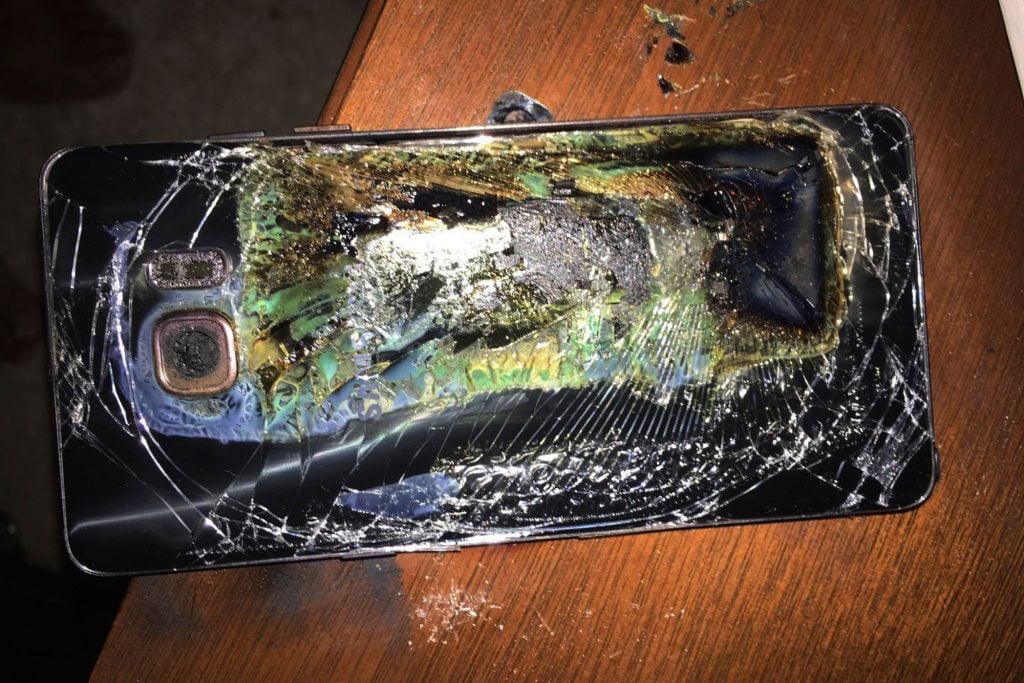
State-of-the-art mechanical characterization of pure lithium metal, performed at submicron-length scales, provides signifcant physical insight into critical factors that limit the performance of next generation energy storage devices.
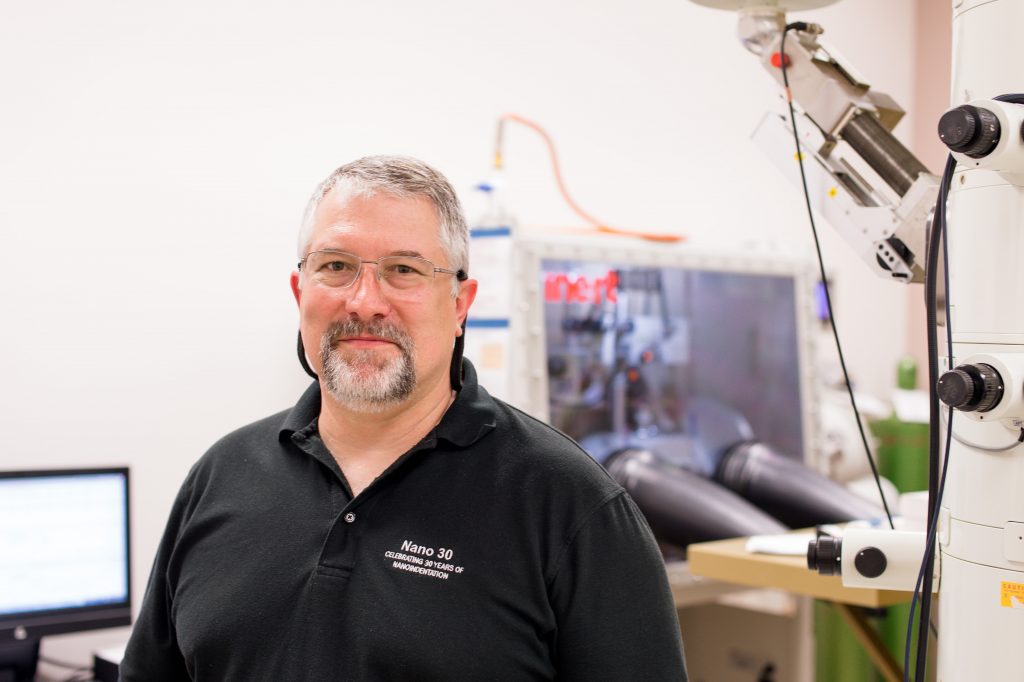
Compared to competing technology platforms, a pure lithium anode potentially offers the highest possible level of volumetric and gravimetric energy density. Gradual loss of lithium over the cycle life of a battery prevents the full fruition of this energy technology.
Michigan Tech researchers Erik Herbert, Stephen Hackney, and their collaborators at Oak Ridge National Laboratory and the University of Michigan are investigating the behavior of a lithium anode accessed through, and protected by, polycrystalline superionic solid electrolytes. Their goals: Mitigate the loss of lithium; prevent dangerous side reactions; and enable safe, long-term, and high-rate cycling performance.
“We want to maintain efficient cycling of lithium in a battery over many cycles, something that’s never been done before,” says Herbert. “The fundamental challenge is figuring out how to maintain a coherent interface between the lithium anode and the solid electrolyte. Defects formed in the lithium during cycling determine the stability and resistivity of the interface. Once we see how that happens, it will reveal design rules necessary to successfully fabricate the solid electrolyte, and the battery packaging.”
The team is launching parallel efforts to address these issues. Herbert, for his part, wants to learn exactly how lithium is consumed on a nanoscale level, in real time. “We want to know why the interface becomes increasingly resistive with cycling, how the electrolyte eventually fails, how defects in the lithium migrate, agglomerate, or anneal with further cycling or time, and whether softer electrolytes can be used without incursion of metallic lithium into the electrolyte,” he says. “We also want to learn how processing and fabrication affect interface performance.”
“We want to maintain efficient cycling of lithium in a battery over many cycles, something that’s never been done before.”
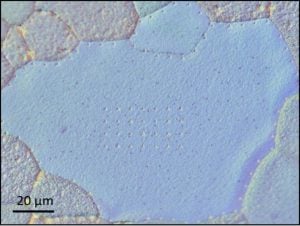
To answer these questions, Herbert conducts nano-indentation studies on vapor-deposited lithium films, various sintered solid electrolytes, and lithium films in fully functional solid-state batteries.
“The data from these experiments directly enable exam-ination of the complex coupling between lithium’s micro-structure, its defects, and its mechanical behavior,” says Herbert. “So far we’ve gained a better understanding of the mechanisms lithium utilizes to manage pressure (stress) as a function of strain, strain rate, temperature, defect structure, microstructural length scale, and in-operando cycling of the battery.”
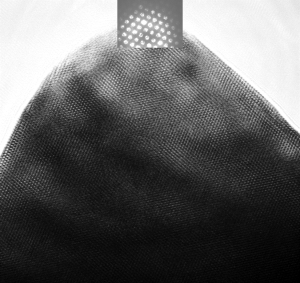
Atomic resolution image of a spinel intergrowth lithium ion battery electrode particle and associated convergent beam electron diffraction pattern. The ordered dots all over the black triangle (the particle) are atomic columns, with a convergent beam electron diffraction pattern in white at the top.
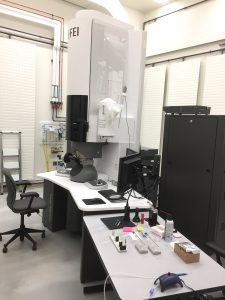
Michigan Tech’s FEI 200kV Titan Themis Scanning Transmission Electron Microscope (S-TEM) positions Michigan Tech faculty on the leading edge of new imaging capability for structural and chemical analysis at the nano-scale.