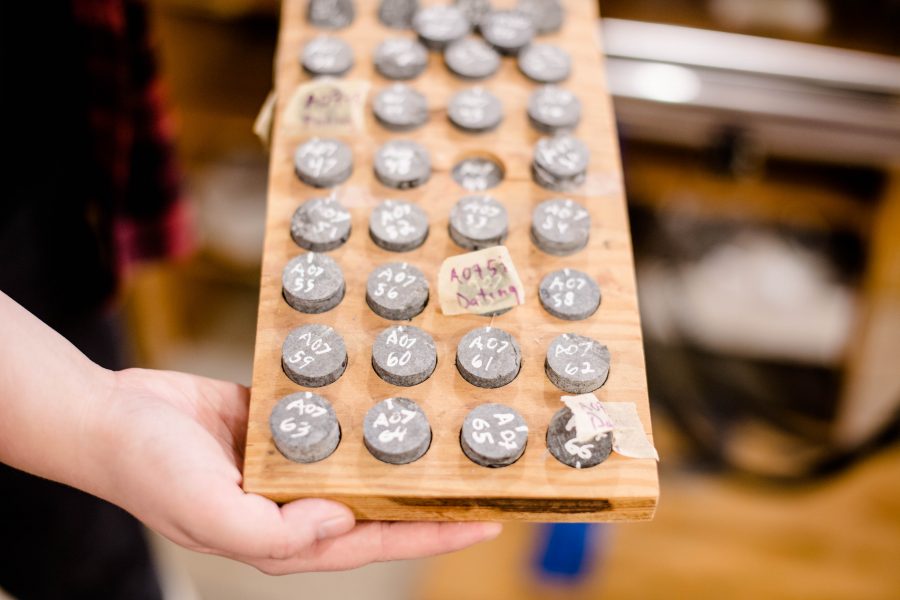
Although it makes up about seven-eighths of the Earth’s history, the Precambrian time period is far from figured out. Key questions remain unanswered.
The Precambrian—the first four billion years of Earth history—was a time of many critical transitions in Earth systems, including oxygenation of the atmosphere and emergence of life. But many of these processes, and the links between them, are poorly understood.
Data can be obtained from fossil magnetism—some rocks record the Earth’s magnetic field that existed at the time of their formation. However, for very old rocks (billions of years old) the conventional methods of obtaining fossil magnetism do not work well.
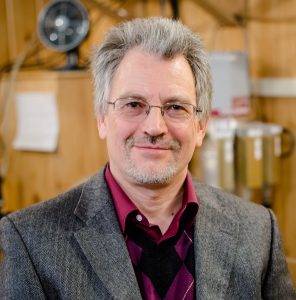
Michigan Tech Professor of Geophysics, Aleksey Smirnov, seeks to substantially increase the amount of reliable data on the Precambrian field. Smirnov investigates the fossil magnetism of well-dated igneous rocks from around the globe using new and experimental processes to help fill in the blanks. His work on the early magnetic field history is supported by several National Science Foundation grants including a National Science Foundation CAREER award.
“Deciphering the early history of our planet, the early history of its geomagnetic field, represents one of the great challenges in Earth science,” says Smirnov. “Available data are scarce, and key questions remain unanswered. For instance we still don’t know how and when the Earth’s geomagnetic field began.”
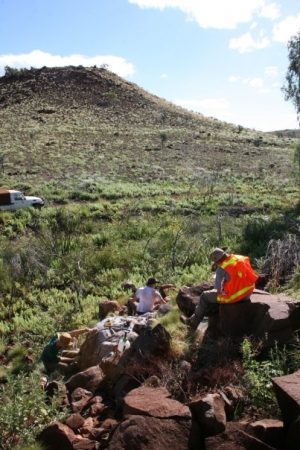
drill rock samples in the Zebra Hill region, Pilbara Craton, Western Australia.
“How did the geomagnetic field evolve at early stages? How did it interact with the biosphere, and other Earth system components—these are all largely unanswered questions. There is also disagreement on the age of the solid inner core, ranging between 0.5 and 2.5 billion years,” note Smirnov.
Scientists largely believe the Earth’s intrinsic magnetic field is generated and maintained by convective flow in the Earth’s fluid outer core, called the geodynamo.
Smirnov’s research has broad implications for Earth science including a better understanding of the workings and age of the geodynamo.
“Crystallization of the inner core may have resulted in a dramatic increase of the geomagnetic field strength preceded by a period of an unusually weak and unstable field,” he explains. “If we observe this behavior in the paleomagnetic record, we will have a much better estimate of the inner core age and hence a better constrained thermal history of our planet.”
Knowing the strength and stability of the early geomagnetic field is also crucial to understanding the causative links between the magnetic field and modulating the evolution of atmosphere and biosphere,” notes Smirnov.
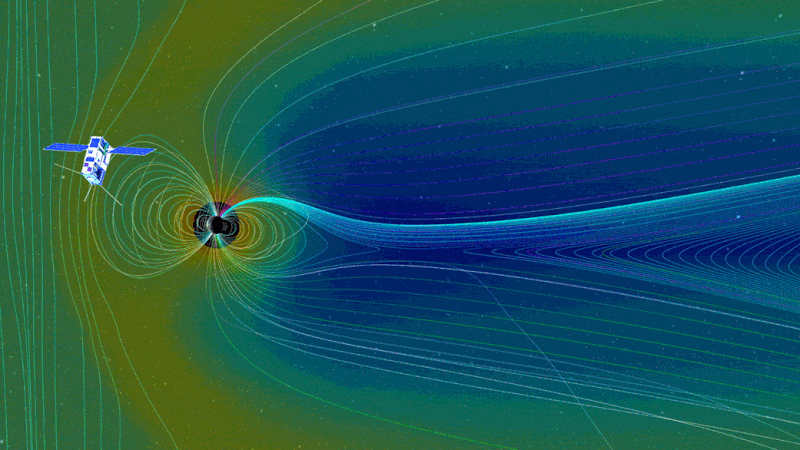
Today, the Earth’s magnetic field protects the atmosphere and life from solar and cosmic radiation. “Before the inner core formation, the geodynamo could have produced a much weaker and less stable magnetic field. An attendant weaker magnetic shielding would allow a much stronger effect of solar radiation on life evolution and atmospheric chemistry.”
Both graduate and undergraduate students work with Smirnov to conduct research, logging hours in his Earth and Environmental Magnetism Lab, traveling the world to collect specimens.
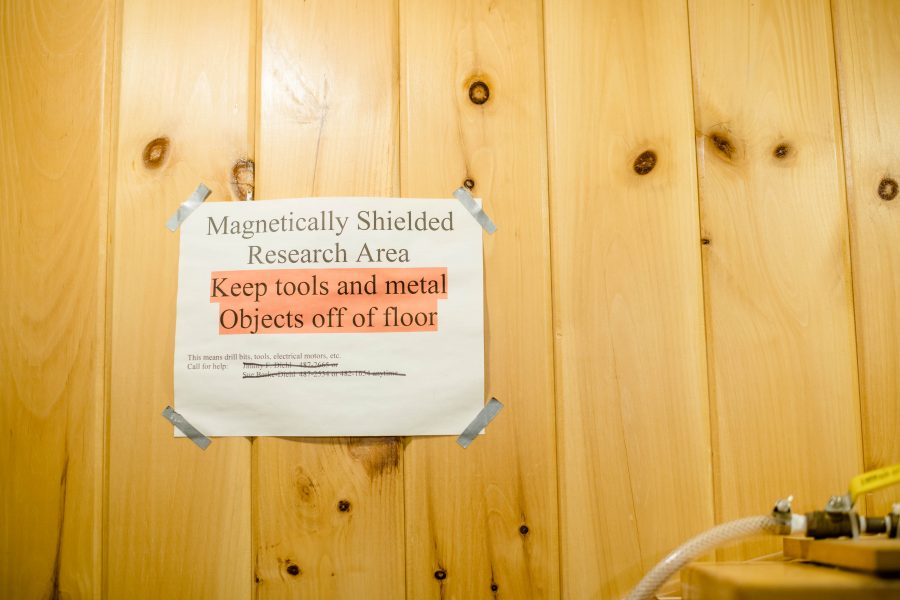
“The primary (useful) magnetizations recorded by ancient rocks are usually very weak and are often superimposed by later (parasitic, secondary) magnetizations,” Smirnov explains. “In order to get to the primary magnetization, we have to remove the secondary magnetizations by incremental heatings of the samples in our specialized paleomagnetic furnaces. The heatings must be done in a zero magnetic field environment. This is one reason why we have the shielded room, which was specially built for our paleomagnetic lab. There are other shielded rooms around the country, but ours is the only one at Michigan Tech,” he notes.
“The second reason for having our instruments in the shielded room is that the magnetizations we measure are weak and our instruments are so sensitive that the Earth’s magnetic field can interfere with our measurements. In fact, in addition to the shielded room, each instrument inside has an additional magnetic shielding.”
Note that the shielded room was built before I came, by my predecessors Profs Jimmy Diehl and Sue Beske-Diehl.
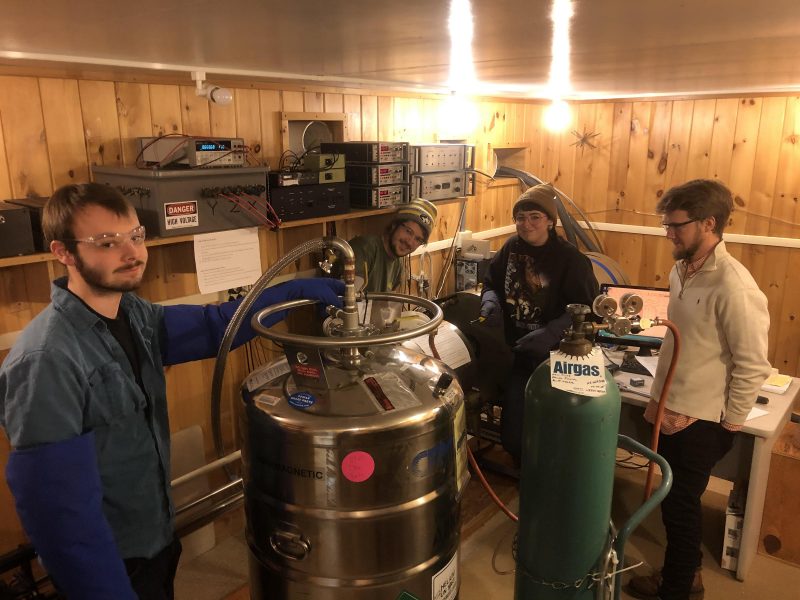
On one month-long trip to the Pilbara Craton in northwest Western Australia, Smirnov and a student gathered 900 samples of well preserved, 2.7 to 3.5 billion year old Precambrian rocks.
Smirnov stepped into the role of chair of the Department of Geological and Mining Engineering and Sciences last fall, but that won’t keep him too far from his research. “Any interested student should feel free to get in touch to learn more about research positions,” he says.
Investigations in Smirnov’s lab are not limited to the ancient field. Other interests include the application of magnetic methods for hydrocarbon exploration, magnetic mineralogy, magnetism of meteorites, biomagnetism, and plate tectonics.
Learn more
Aleksey Smirnov is the new Chair of Geological and Mining Engineering and Sciences
Clues To Earth’s Ancient Core